Ettingshausen effect: Difference between revisions
en>FrescoBot m Bot: link syntax/spacing |
en>Myasuda m avoid redirect |
||
Line 1: | Line 1: | ||
In the [[solid-state physics]] of [[semiconductor]]s, '''carrier generation''' and '''recombination''' are processes by which mobile [[charge carrier]]s ([[electron]]s and [[electron hole]]s) are created and eliminated. Carrier generation and recombination processes are fundamental to the operation of many [[optoelectronics|optoelectronic]] [[semiconductor device]]s, such as [[photodiode]]s, [[Light-emitting diode|LED]]s and [[laser diode]]s. They are also critical to a full analysis of [[p-n junction]] devices such as [[bipolar junction transistor]]s and p-n junction [[diode]]s. | |||
The '''electron–hole pair''' is the fundamental unit of generation and recombination, corresponding to an electron transitioning between the valence band and the conduction band. | |||
==Band structure== | |||
Like other solids, semiconductor materials have [[electronic band structure]] determined by the crystal properties of the material. The actual energy distribution among the electrons is described by the [[Fermi level]] and the [[temperature]] of the electrons. At [[absolute zero]] temperature, all of the electrons have energy below the Fermi level; but at non-zero temperatures the energy levels are filled following a Boltzmann distribution. | |||
[[Image:Electronic band diagram.svg|thumb|375px|Electronic band structure of a semiconductor material.]]In semiconductors the Fermi level lies in the middle of a ''forbidden band'' or [[band gap]] between two ''allowed bands'' called the ''[[valence band]]'' and the ''[[conduction band]]''. The valence band, immediately below the forbidden band, is normally very nearly completely occupied. The conduction band, above the Fermi level, is normally nearly completely empty. Because the valence band is so nearly full, its electrons are not mobile, and cannot flow as electrical current. | |||
However, if an electron in the valence band acquires enough energy to reach the conduction band, it can flow freely among the nearly empty conduction band energy states. Furthermore it will also leave behind an electron hole that can flow as current exactly like a physical charged particle. Carrier ''generation'' describes processes by which electrons gain energy and move from the valence band to the conduction band, producing two mobile carriers; while ''recombination'' describes processes by which a conduction band electron loses energy and re-occupies the energy state of an electron hole in the valence band. | |||
In a material at [[thermal equilibrium]] generation and recombination are balanced, so that the net [[charge carrier]] density remains constant. The equilibrium carrier density that results from the balance of these interactions is predicted by [[thermodynamics]]. The resulting probability of occupation of energy states in each energy band is given by [[Fermi–Dirac statistics]]. | |||
===Generation and recombination processes=== | |||
Carrier generation and recombination result from interaction between [[electron]]s and other carriers, either with the [[crystal structure|lattice]] of the material, or with optical [[photon]]s. As the electron moves from one energy band to another, its gained or lost energy must take some other form, and the form of energy distinguishes various types of generation and recombination: | |||
[[File:diffusion_center.gif|thumb|350px|right|The following image shows change in excess carriers being generated (green:electrons and purple:holes) with increasing light intensity (Generation rate /cm3) at the center of an intrinsic semiconductor bar. Electrons have higher diffusion constant than holes leading to fewer excess electrons at the center as compared to holes.]] | |||
=== Shockley–Read–Hall (SRH) process === | |||
The electron in transition between bands passes through a new energy state created within the band gap by an impurity in the lattice (Localized State). The localized state can absorb differences in [[momentum]] between the carriers, and so this process is the dominant generation and recombination process in [[silicon]] and other [[direct and indirect band gaps|indirect bandgap]] materials. It can also dominate in [[direct and indirect band gaps|direct bandgap]] materials under conditions of very low [[charge carrier density|carrier densities]] (very low level injection). The energy is exchanged in the form of lattice vibration, or a [[phonon]] exchanging thermal energy with the material. The impurities create [[energy level]]s within the [[band gap]], forming [[deep-level trap]]s. | |||
=== Photon exchange === | |||
During ''radiative recombination'', a form of [[spontaneous emission]], a [[photon]] is emitted with the wavelength corresponding to the energy released. This effect is the basis of [[light-emitting diode|LEDs]]. Because the photon carries relatively little [[momentum]], radiative recombination is significant only in [[direct bandgap]] materials. | |||
When photons are present in the material, they can either be [[absorption (electromagnetic radiation)|absorbed]], generating a pair of free carriers, or they can ''stimulate'' a recombination event, resulting in a generated photon with similar properties to the one responsible for the event. Absorption is the active process in [[photodiode]]s, [[solar cell]]s, and other semiconductor [[photodetector]]s, while [[stimulated emission]] is responsible for laser action in [[laser diode]]s. | |||
=== Auger recombination === | |||
The energy is given to a third carrier, which is excited to a higher energy level without moving to another energy band. After the interaction, the third carrier normally loses its excess energy to thermal vibrations. Since this process is a three-particle interaction, it is normally only significant in non-equilibrium conditions when the carrier density is very high. The [[Auger effect]] process is not easily produced, because the third particle would have to begin the process in the unstable high-energy state. | |||
The Auger recombination can be calculated from the equation : | |||
: <math>U_{Aug} = \Gamma_n \,n(np-n_i^2) + \Gamma_p \,p(np-n_i^2)</math> | |||
==== Auger recombination in LEDs ==== | |||
The mechanism causing [[Light-emitting diode#Efficiency droop|LED efficiency droop]] was identified in 2007 as Auger recombination, which was taken with mixed reaction.<ref name=stevenson>Stevenson, Richard (August 2009) [http://www.spectrum.ieee.org/semiconductors/optoelectronics/the-leds-dark-secret The LED’s Dark Secret: Solid-state lighting won't supplant the lightbulb until it can overcome the mysterious malady known as droop]. IEEE Spectrum</ref> In 2013, a study conclusively identified Auger recombination at the cause of efficiency droop.<ref>{{cite web|last=Justin Iveland, Lucio Martinelli, Jacques Peretti, James S. Speck, Claude Weisbuch.|title=Cause of LED Efficiency Droop Finally Revealed|url=http://www.sciencedaily.com/releases/2013/04/130423102328.htm|work=Physical Review Letters, 2013|publisher=Science Daily|accessdate=23 April 2013}}</ref> | |||
==References== | |||
<references /> | |||
* N.W. Ashcroft and N.D. Mermin, ''Solid State Physics'', Brooks Cole, 1976 | |||
==External links== | |||
* [http://www.pvlighthouse.com.au/calculators/Recombination%20calculator/Recombination%20calculator.aspx PV Lighthouse Recombination Calculator] | |||
* [http://www.pvlighthouse.com.au/calculators/Band%20gap%20calculator/Band%20gap%20calculator.aspx PV Lighthouse Band Gap Calculator] | |||
[[Category:Condensed matter physics]] | |||
[[Category:Optoelectronics]] | |||
[[Category:Charge carriers]] |
Revision as of 05:45, 7 December 2013
In the solid-state physics of semiconductors, carrier generation and recombination are processes by which mobile charge carriers (electrons and electron holes) are created and eliminated. Carrier generation and recombination processes are fundamental to the operation of many optoelectronic semiconductor devices, such as photodiodes, LEDs and laser diodes. They are also critical to a full analysis of p-n junction devices such as bipolar junction transistors and p-n junction diodes.
The electron–hole pair is the fundamental unit of generation and recombination, corresponding to an electron transitioning between the valence band and the conduction band.
Band structure
Like other solids, semiconductor materials have electronic band structure determined by the crystal properties of the material. The actual energy distribution among the electrons is described by the Fermi level and the temperature of the electrons. At absolute zero temperature, all of the electrons have energy below the Fermi level; but at non-zero temperatures the energy levels are filled following a Boltzmann distribution.
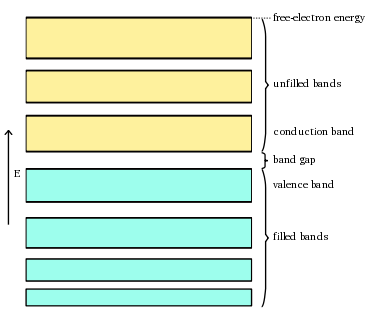
In semiconductors the Fermi level lies in the middle of a forbidden band or band gap between two allowed bands called the valence band and the conduction band. The valence band, immediately below the forbidden band, is normally very nearly completely occupied. The conduction band, above the Fermi level, is normally nearly completely empty. Because the valence band is so nearly full, its electrons are not mobile, and cannot flow as electrical current.
However, if an electron in the valence band acquires enough energy to reach the conduction band, it can flow freely among the nearly empty conduction band energy states. Furthermore it will also leave behind an electron hole that can flow as current exactly like a physical charged particle. Carrier generation describes processes by which electrons gain energy and move from the valence band to the conduction band, producing two mobile carriers; while recombination describes processes by which a conduction band electron loses energy and re-occupies the energy state of an electron hole in the valence band.
In a material at thermal equilibrium generation and recombination are balanced, so that the net charge carrier density remains constant. The equilibrium carrier density that results from the balance of these interactions is predicted by thermodynamics. The resulting probability of occupation of energy states in each energy band is given by Fermi–Dirac statistics.
Generation and recombination processes
Carrier generation and recombination result from interaction between electrons and other carriers, either with the lattice of the material, or with optical photons. As the electron moves from one energy band to another, its gained or lost energy must take some other form, and the form of energy distinguishes various types of generation and recombination:

Shockley–Read–Hall (SRH) process
The electron in transition between bands passes through a new energy state created within the band gap by an impurity in the lattice (Localized State). The localized state can absorb differences in momentum between the carriers, and so this process is the dominant generation and recombination process in silicon and other indirect bandgap materials. It can also dominate in direct bandgap materials under conditions of very low carrier densities (very low level injection). The energy is exchanged in the form of lattice vibration, or a phonon exchanging thermal energy with the material. The impurities create energy levels within the band gap, forming deep-level traps.
Photon exchange
During radiative recombination, a form of spontaneous emission, a photon is emitted with the wavelength corresponding to the energy released. This effect is the basis of LEDs. Because the photon carries relatively little momentum, radiative recombination is significant only in direct bandgap materials.
When photons are present in the material, they can either be absorbed, generating a pair of free carriers, or they can stimulate a recombination event, resulting in a generated photon with similar properties to the one responsible for the event. Absorption is the active process in photodiodes, solar cells, and other semiconductor photodetectors, while stimulated emission is responsible for laser action in laser diodes.
Auger recombination
The energy is given to a third carrier, which is excited to a higher energy level without moving to another energy band. After the interaction, the third carrier normally loses its excess energy to thermal vibrations. Since this process is a three-particle interaction, it is normally only significant in non-equilibrium conditions when the carrier density is very high. The Auger effect process is not easily produced, because the third particle would have to begin the process in the unstable high-energy state.
The Auger recombination can be calculated from the equation :
Auger recombination in LEDs
The mechanism causing LED efficiency droop was identified in 2007 as Auger recombination, which was taken with mixed reaction.[1] In 2013, a study conclusively identified Auger recombination at the cause of efficiency droop.[2]
References
- ↑ Stevenson, Richard (August 2009) The LED’s Dark Secret: Solid-state lighting won't supplant the lightbulb until it can overcome the mysterious malady known as droop. IEEE Spectrum
- ↑ Template:Cite web
- N.W. Ashcroft and N.D. Mermin, Solid State Physics, Brooks Cole, 1976