Isoperimetric dimension: Difference between revisions
en>Josephorourke |
en>Addbot m Bot: Migrating 1 interwiki links, now provided by Wikidata on d:q3707726 |
||
Line 1: | Line 1: | ||
[[File:FELIX.jpg|265px|thumb|The free-electron laser ''FELIX'' at the FOM Institute for Plasma Physics Rijnhuizen, [[Nieuwegein]], The Netherlands.]] | |||
A '''free-electron laser''' (FEL), is a type of [[laser]] that shares the same [[optics|optical]] properties as conventional lasers such as emitting a [[charged particle beam|beam]] consisting of [[coherence (physics)|coherent]] [[electromagnetic radiation|electromagnetic]] [[radiation]] that can reach high [[power (physics)|power]], but that uses some very different operating principles to form the beam. Unlike [[gas laser|gas]]-, [[liquid]]-, or [[solid-state laser|solid-state]] lasers such as [[diode laser]]s, in which electrons are excited in bound atomic or molecular states, free-electron lasers use a [[relativistic electron beam]] that moves freely through a magnetic structure,<ref name="huang">{{Cite doi|10.1103/PhysRevSTAB.10.034801}}</ref> hence the term ''free electron'' as the [[active laser medium|lasing medium]].<ref>{{cite web |title=Duke University Free-Electron Laser Laboratory| url=http://www.fel.duke.edu/| accessdate=2007-12-21}}</ref>{{failed verification|date=October 2013}} The free-electron laser has the widest [[frequency]] range of any laser type, and can be widely tunable,<ref>[[F. J. Duarte]] (Ed.), ''Tunable Lasers Handbook'' (Academic, New York, 1995) Chapter 9.</ref> currently ranging in [[wavelength]] from [[microwave]]s, through [[terahertz radiation]] and [[infrared]], to the [[visible spectrum]], [[ultraviolet]], and [[X-ray]].<ref>{{cite web |title=New Era of Research Begins as World's First Hard X-ray Laser Achieves "First Light" |publisher=SLAC National Accelerator Laboratory |date=April 21, 2009 |accessdate=2013-11-06 | |||
|url=http://home.slac.stanford.edu/pressreleases/2009/20090421.htm}}</ref> | |||
[[Image:FEL principle.png|400px|thumb|right|Schematic representation of an [[undulator]], at the core of a free-electron laser.]] | |||
Free-electron lasers were invented by [[John Madey]] in 1976 at [[Stanford University]]. The work emanates from research done by [[Hans Motz]] and his coworkers, who built an [[undulator]] at [[Stanford]] in 1953,<ref>{{Cite doi|10.1063.2F1.1700002}}</ref><ref>{{Cite doi|10.1063/1.1721389}}</ref> using the [[Wiggler (synchrotron)|wiggler]] magnetic configuration which is at the heart of a free electron laser. Madey used a 24 MeV electron beam and 5 m long wiggler to amplify a signal. Soon afterward, other laboratories with accelerators started developing such lasers. | |||
==Beam creation== | |||
[[File:Undulator.FELIX.jpg|265px|thumb|The undulator of ''FELIX''.]] | |||
To create an FEL, a beam of [[electron]]s is accelerated to almost the [[speed of light]]. The beam passes through an undulator, a side to side [[magnetic field]] produced by a periodic arrangement of [[magnet]]s with alternating [[dipole|poles]] across the beam path. The general direction of the beam is called the longitudinal direction, and the direction across the beam path is called transverse. This array of magnets is commonly known as an [[undulator]] in the light source community, or a [[wiggler (synchrotron)|wiggler]] in the FEL community,{{citation needed|date=October 2013}} because it forces the electrons in the beam to wiggle transversely along a sinusoidal path about the axis of the undulator. | |||
The transverse acceleration of the electrons across this path results in the release of [[photons]] ([[synchrotron radiation]]), which are monochromatic but still incoherent, | |||
because the electromagnetic waves from randomly distributed electrons interfere constructively and destructively in time, and the resulting radiation power scales linearly with the number of electrons. If an external laser is provided or if the synchrotron radiation becomes sufficiently strong, the transverse electric field of the radiation beam interacts with the transverse electron current created by the sinusoidal wiggling motion, causing some electrons to gain and others to lose energy to the optical field.{{clarify|date=October 2013}} | |||
This energy modulation evolves into electron density (current) modulations with a period of one optical wavelength. The electrons are thus bunched into little clumps, called ''microbunches'', separated by one optical wavelength along the axis. Whereas conventional undulators would cause the electrons to radiate independently, the radiation emitted by the microbunched electrons are in phase, and the fields add together [[coherence (physics)|coherently]]. | |||
The FEL radiation intensity grows, causing additional microbunching of the electrons, which continue to radiate in phase with each other.<ref>Feldhaus et al., J. Phys. B: At. Mol. Opt. Phys. 38 (2005) S799–S819</ref> This process continues until the electrons are completely microbunched and the radiation reaches a saturated power several orders of magnitude higher than that of the undulator radiation. | |||
The wavelength of the radiation emitted can be readily tuned by adjusting the energy of the electron beam or the magnetic field strength of the undulators. | |||
FELs are relativistic machines. The wavelength of the emitted radiation, <math>\lambda_r</math>, is given by <ref>{{Cite doi|10.1103/RevModPhys.74.685}}</ref> | |||
:<math>\lambda_r = \frac{\lambda_u}{2 \gamma^2}(1+K^2)</math> , | |||
or when the wiggler strength parameter K, discussed below, is small | |||
:<math>\lambda_r \propto \frac{\lambda_u}{2 \gamma^2}</math> , | |||
where <math>\lambda_u</math> is the undulator wavelength (the spatial period of the magnetic field), <math>\gamma</math> is the relativistic [[Lorentz factor]] and the proportionality constant depends on the undulator geometry and is of the order of 1. | |||
This formula can be understood as a combination of two relativistic effects. Imagine you are sitting on an electron passing through the undulator. Due to [[Lorentz contraction]] the undulator is shortened by a <math>\gamma</math> factor and the electron experiences much shorter undulator wavelength <math>\lambda_u/\gamma</math>. However, the radiation emitted at this wavelength is observed in the laboratory frame of reference and the [[relativistic Doppler effect]] brings the second <math>\gamma</math> factor to the above formula. Rigorous derivation from Maxwell's equations gives the divisor of 2 and the proportionality constant. In an x-ray FEL the typical undulator wavelength of 1 cm is transformed to x-ray wavelengths on the order of 1 nm by <math>\gamma</math> ≈ 2000, i.e. the electrons have to travel with the speed of 0.9999998''c''. | |||
===Wiggler strength parameter K=== | |||
K, a [[dimensionless]] parameter, tells the wiggler strength as the relationship between the length of a period and the radius of bend,<ref>{{cite web |title=WIGGLER |author=Robert Soliday |date=2006-09-05 | |||
|publisher=Argon National laboratory | |||
|accessdate=2013-10-18}}</ref> | |||
:<math>K = \frac{\gamma \lambda_u}{2 \pi \rho }</math> | |||
where <math>\rho</math> is the bending radius. | |||
===Quantum effects=== | |||
In most cases, the theory of [[classical electromagnetism]] adequately accounts for the behavior of free electron lasers.<ref name="fain">{{Cite doi|10.1364/JOSAB.4.000078}}</ref> For sufficiently short wavelengths, [[quantum mechanics|quantum effects]] of electron recoil and [[Electronic_noise#Shot_noise|shot noise]] may have to be considered.<ref>{{Cite doi|10.1063/1.34633}}</ref> | |||
==Large facilities required== | |||
Today, a free-electron laser requires the use of an electron [[particle accelerator|accelerator]] with its associated shielding, as accelerated electrons can be a radiation hazard, if not properly contained. These accelerators are typically powered by [[klystron]]s, which require a high voltage supply. The electron beam must be maintained in a [[vacuum]] which requires the use of numerous [[vacuum pump]]s along the beam path. While this equipment is bulky and expensive, free-electron lasers can achieve very high peak powers, and the tunability of FELs makes them highly desirable in many disciplines, including chemistry, structure determination of molecules in biology, [[medical diagnosis]], and [[nondestructive testing]]. | |||
==X-ray laser without mirrors== | |||
The lack of suitable [[mirror]]s in the extreme [[ultraviolet]] and [[x-ray]] regimes prevents the operation of a FEL oscillator; consequently, there must be suitable amplification over a single pass of the electron beam through the undulator to make the FEL worthwhile. X-ray free electron lasers use long [[undulator]]s. The underlying principle of the intense pulses from the X-ray laser lies in the principle of [[self-amplified stimulated emission]] (SASE), which leads to the [[microbunching]] of the electrons. Initially all electrons are distributed evenly and they emit incoherent spontaneous radiation only. Through the interaction of this radiation and the electrons' [[oscillation]]s, they drift into microbunches separated by a distance equal to one radiation wavelength. Through this interaction, all electrons begin emitting coherent radiation in phase. In other words, all emitted radiation can reinforce itself perfectly whereby wave crests and wave troughs are always superimposed on one another in the best possible way. This results in an exponential increase of emitted radiation power, leading to high beam intensities and laser-like properties.<ref name=XFEL>{{cite web |title=XFEL information webpages |url=http://xfelinfo.desy.de/en/start/2/index.html |accessdate=2007-12-21}}</ref> Examples of facilities operating on the SASE FEL principle include the Free electron LASer ([[DESY#Particle Accelerators, Facilities and Experiments at DESY|FLASH]]) in Hamburg, the [[Linac Coherent Light Source]] (LCLS) at the [[SLAC National Accelerator Laboratory]], the [[European x-ray free electron laser]] (XFEL) in Hamburg, the [[SPring-8]] Compact SASE Source (SCSS), the [[SwissFEL]] at the [[Paul Scherrer Institute]] (Switzerland) and, as of 2011, the SACLA at the [[RIKEN]] Harima Institute in Japan. | |||
==Self seeding== | |||
One problem with SASE FELs is the lack of [[time|temporal]] coherence due to a [[shot noise|noisy]] startup process. To avoid this, one can "seed" an FEL with a [[laser]] tuned to the resonance of the FEL. Such a temporally coherent seed can be produced by more conventional means, such as by [[high-harmonic generation]] (HHG) using an optical laser pulse. This results in coherent [[:wikt:amplification|amplification]] of the input signal; in effect, the output laser quality is characterized by the seed. While HHG seeds are available at [[wavelength]]s down to the extreme ultraviolet, seeding is not feasible at [[x-ray]] wavelengths due to the lack of conventional x-ray lasers. | |||
In late 2010, in Italy, the seeded-FEL source FERMI@Elettra <ref>http://www.elettra.trieste.it/FERMI/</ref> has started commissioning, at the Sincrotrone Trieste Laboratory. FERMI@Elettra is a single-pass FEL user-facility covering the wavelength range from 100 nm (12 eV) to 10 nm (124 eV), located next to the third-generation synchrotron radiation facility ELETTRA in Trieste, Italy. The advent of femtosecond lasers has revolutionized many areas of science from solid state physics to biology. This new research frontier of ultra-fast VUV and X-ray science drives the development of a novel source for the generation of femtosecond pulses. | |||
In 2012, scientists working on the LCLS overcame the seeding limitation for x-ray wavelengths by self-seeding the laser with its own beam after being filtered through a diamond monochromator. The resulting intensity and monochromaticity of the beam are unprecedented and will allow new experiments to be conducted involving manipulating atoms and imaging molecules. Other labs around the world are incorporating the technique into their equipment.<ref name="nature"> | |||
{{Cite doi|10.1038/nphoton.2012.180}}</ref><ref>{{cite web | |||
|title='Self-seeding' promises to speed discoveries, add new scientific capabilities |date=August 13, 2012 |publisher=SLAC National Accelerator Laboratory | |||
|url=https://news.slac.stanford.edu/press-release/worlds-most-powerful-x-ray-laser-beam-refined-scalpel-precision |accessdate=2013-11-06}}</ref> | |||
==Medical uses== | |||
Research by Dr. Glenn Edwards and colleagues at [[Vanderbilt University]]'s FEL Center in 1994 found that soft tissues like skin, cornea, and brain tissue could be cut, or ablated, using [[infrared]] FEL wavelengths around 6.45 micrometres with minimal collateral damage to adjacent tissue.<ref>Glenn Edwards et al., Nature 371 (1994) 416-419</ref><ref>{{cite web |title=Laser light from Free-Electron Laser used for first time in human surgery |url=http://www.vanderbilt.edu/News/register/Jan10_00/story5.html |accessdate=2010-11-06}}</ref> This led to further research and eventually surgeries on humans, the first ever using a free-electron laser. Starting in 1999, and using the Keck foundation funded FEL operating rooms at the Vanderbilt FEL Center, Dr. Michael Copeland and Dr. Pete Konrad of Vanderbilt performed three surgeries in which they resected [[meningioma]] [[brain tumor]]s.<ref>Glenn S. Edwards et al., Rev. Sci. Instrum. 74 (2003) 3207</ref> Beginning in 2000, Dr. Karen Joos and Dr. Louise Mawn performed five surgeries involving the cutting of a window in the sheath of the optic nerve, to test the efficacy for optic nerve sheath fenestration.<ref>Karen M. Joos et al., in Proc. of SPIE 4611 (2002) 81-85, eds. Manns, Soederberg, and Ho.</ref> These eight surgeries went as expected with results consistent with the routine standard of care and with the added benefit of laser surgery and minimal collateral damage. A review of FELs for medical uses is given in the 1st edition of Tunable Laser Applications.<ref>F. J. Duarte (Ed.), ''Tunable Laser Applications'', 1st Ed. (Marcel-Dekker, New York, 1995) Chapter 6. | |||
</ref> | |||
Since these successful results, there have been several efforts to build small, clinical lasers tunable in the 6 to 7 micrometre range with pulse structure and energy to give minimal collateral damage in soft tissue.{{Citation needed|date=November 2010}} At Vanderbilt, there exists a Raman shifted system pumped by an Alexandrite laser.<ref>{{cite web |title=Efficiency and Plume Dynamics for Mid-IR Laser Ablation of Cornea |url=http://meetings.aps.org/link/BAPS.2009.MAR.T27.6 |accessdate=2010-11-06 |date=2009-03-18}}</ref> | |||
At the 2006 annual meeting of the American Society for Laser Medicine and Surgery (ASLMS), [[R. Rox Anderson|Dr. Rox Anderson]] of the Wellman Laboratory of Photomedicine of [[Harvard Medical School]] and [[Massachusetts General Hospital]] reported on the possible medical application of the free-electron laser in melting fats without harming the overlying skin.<ref>{{cite news |title=BBC health |url=http://news.bbc.co.uk/1/hi/health/4895148.stm |accessdate=2007-12-21 | date=2006-04-10 |work=BBC News}}</ref> It was reported that at [[infrared]] [[wavelength]]s, water in tissue was heated by the laser, but at wavelengths corresponding to 915, 1210 and 1720 [[nanometer|nm]], subsurface [[lipid]]s were differentially heated more strongly than water. The possible applications of this selective photothermolysis (heating tissues using light) include the selective destruction of sebum lipids to treat [[acne vulgaris|acne]], as well as targeting other lipids associated with [[cellulite]] and body fat as well as fatty plaques that form in arteries which can help treat [[atherosclerosis]] and [[heart disease]].<ref>{{cite web |title=Dr Rox Anderson treatment |url=http://www.physorg.com/news63806610.html |accessdate=2007-12-21}}</ref> | |||
==Military uses== | |||
FEL technology is being evaluated by the [[US Navy]] as a good candidate for an anti aircraft and missile [[directed-energy weapon]]. Significant progress is being made in raising FEL power levels (the [[Thomas Jefferson National Accelerator Facility]]'s FEL has demonstrated over 14 kW)<ref>{{cite web | title=Jefferson Lab FEL |url=http://www.jlab.org/fel/ |accessdate=2009-06-08}}</ref> and it should be possible to build compact multi-megawatt class FEL weapons.<ref>{{cite web |title=Airborne megawatt class free-electron laser for defense and security |url=http://www.osti.gov/energycitations/product.biblio.jsp?osti_id=841301 |accessdate=2007-12-21}}</ref> On June 9, 2009 the [[Office of Naval Research]] announced it had awarded [[Raytheon]] a contract to develop a 100 kW experimental FEL.<ref>{{cite web |title=Raytheon Awarded Contract for Office of Naval Research's Free Electron Laser Program |url=http://news.prnewswire.com/ViewContent.aspx?ACCT=109&STORY=/www/story/06-09-2009/0005040843&EDATE= |accessdate=2009-06-12}}</ref> On March 18, 2010 Boeing Directed Energy Systems announced the completion of an initial design for U.S. Naval use.<ref>{{cite web |title=Boeing Completes Preliminary Design of Free Electron Laser Weapon System |url=http://boeing.mediaroom.com/index.php?s=43&item=1126 |accessdate=2010-03-29}}</ref> A prototype FEL system has since been demonstrated with a full-power prototype scheduled by 2018.<ref>{{cite news | url=http://www.foxnews.com/scitech/2011/01/20/raygun-breakthrough-revolutionize-naval-power/?test=faces | title=Breakthrough Laser Could Revolutionize Navy's Weaponry | accessdate=2011-01-22 | publisher=Fox News | date=2011-01-20}}</ref> | |||
==See also== | |||
* [[Bremsstrahlung]] | |||
* [[Cyclotron radiation]] | |||
* [[Electron wake]] | |||
* [[Gyrotron]] | |||
* [[International Linear Collider]] | |||
* [[Synchrotron radiation]] | |||
==References== | |||
{{reflist|2}} | |||
==Further reading== | |||
* Madey, John, "Stimulated emission of bremsstrahlung in a periodic magnetic field". J. Appl. Phys. 42, 1906 (1971) | |||
* Madey, John, Stimulated emission of radiation in periodically deflected electron beam, US Patent 38 22 410,1974 | |||
* Boscolo, ''et al.'', "''Free-Electron Lasers and Masers on Curved Paths''". Appl. Phys., (Germany), vol. 19, No. 1, pp. 46–51, May 1979. | |||
* Deacon ''et al.'', "''First Operation of a Free-Electron Laser''". Phys. Rev. Lett., vol. 38, No. 16, Apr. 1977, pp. 892–894. | |||
* Elias, ''et al.'', "''Observation of Stimulated Emission of Radiation by Relativistic Electrons in a Spatially Periodic Transverse Magnetic Field''", Phys. Rev. Lett., 36 (13), 1976, p. 717. | |||
* Gover, "''Operation Regimes of Cerenkov-Smith-Purcell Free Electron Lasers and T. W. Amplifiers''". Optics Communications, vol. 26, No. 3, Sep. 1978, pp. 375–379. | |||
* Gover, "''Collective and Single Electron Interactions of Electron Beams with Electromagnetic Waves and Free Electrons Lasers''". App. Phys. 16 (1978), p. 121. | |||
* "''The FEL Program at Jefferson Lab''" [http://www.jlab.org/fel] | |||
* {{Cite journal | first = Charles | last = Brau | title = Free-Electron Lasers | place = Boston | publisher = Academic Press, Inc. | |||
| year = 1990 | postscript = <!--None--> }} | |||
*Paolo Luchini, Hans Motz, ''Undulators and Free-electron Lasers'', Oxford University Press, 1990. | |||
==External links== | |||
* [http://www.lightsources.org Lightsources.org] | |||
* [http://www.elettra.trieste.it/lightsources/fermi.html FERMI], the new FEL at the [[ELETTRA]] synchrotron in Triest | |||
* [http://sbfel3.ucsb.edu/ UCSB Free-Electron Laser] | |||
* [http://www.nap.edu/books/NI000099/html/ Free-Electron Laser Open Book (National Academies Press)] | |||
* [http://sbfel3.ucsb.edu/www/vl_fel.html The World Wide Web Virtual Library: Free-Electron Laser research and applications] | |||
* [http://www.xfel.eu/ European XFEL] | |||
* [http://fel.web.psi.ch/ PSI SwissFEL] | |||
* [http://www-xfel.spring8.or.jp/ SPring-8 Compact SASE Source] | |||
* [http://www.fz-rossendorf.de/pls/rois/Cms?pOid=10548&pNid=471 Electron beam transport system and diagnostics of the Dresden FEL] | |||
* [http://www.rijnhuizen.nl/research/guthz/felix_felice The Free Electron Laser for Infrared eXperiments FELIX] | |||
* [http://www.vanderbilt.edu/fel/ W. M. Keck Free Electron Laser Center] | |||
* [http://www.jlab.org/fel Jefferson Lab's Free-Electron Laser Program] | |||
* [http://www.newscientist.com/channel/mech-tech/mg18925351.300 Free-Electron Lasers: The Next Generation] by Davide Castelvecchi [[New Scientist]], January 21, 2006 | |||
* [http://www.osti.gov/energycitations/product.biblio.jsp?osti_id=841301 Airborne megawatt class free-electron laser for defense and security] | |||
* [http://www.elettra.trieste.it/FERMI/ FERMI@Elettra Free-Electron Laser Project] | |||
* [http://www.cfel.de Center for Free-Electron Laser Science (CFEL)] | |||
{{DEFAULTSORT:Free-Electron Laser}} | |||
[[Category:Electron beam]] | |||
[[Category:Laser types]] | |||
[[Category:Medical equipment]] | |||
[[Category:Terahertz technology]] | |||
[[Category:Accelerator physics]] |
Revision as of 15:10, 13 March 2013
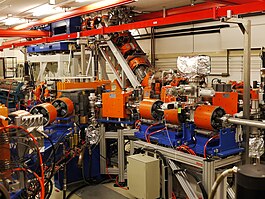
A free-electron laser (FEL), is a type of laser that shares the same optical properties as conventional lasers such as emitting a beam consisting of coherent electromagnetic radiation that can reach high power, but that uses some very different operating principles to form the beam. Unlike gas-, liquid-, or solid-state lasers such as diode lasers, in which electrons are excited in bound atomic or molecular states, free-electron lasers use a relativistic electron beam that moves freely through a magnetic structure,[1] hence the term free electron as the lasing medium.[2]Template:Failed verification The free-electron laser has the widest frequency range of any laser type, and can be widely tunable,[3] currently ranging in wavelength from microwaves, through terahertz radiation and infrared, to the visible spectrum, ultraviolet, and X-ray.[4]

Free-electron lasers were invented by John Madey in 1976 at Stanford University. The work emanates from research done by Hans Motz and his coworkers, who built an undulator at Stanford in 1953,[5][6] using the wiggler magnetic configuration which is at the heart of a free electron laser. Madey used a 24 MeV electron beam and 5 m long wiggler to amplify a signal. Soon afterward, other laboratories with accelerators started developing such lasers.
Beam creation

To create an FEL, a beam of electrons is accelerated to almost the speed of light. The beam passes through an undulator, a side to side magnetic field produced by a periodic arrangement of magnets with alternating poles across the beam path. The general direction of the beam is called the longitudinal direction, and the direction across the beam path is called transverse. This array of magnets is commonly known as an undulator in the light source community, or a wiggler in the FEL community,Potter or Ceramic Artist Truman Bedell from Rexton, has interests which include ceramics, best property developers in singapore developers in singapore and scrabble. Was especially enthused after visiting Alejandro de Humboldt National Park. because it forces the electrons in the beam to wiggle transversely along a sinusoidal path about the axis of the undulator.
The transverse acceleration of the electrons across this path results in the release of photons (synchrotron radiation), which are monochromatic but still incoherent, because the electromagnetic waves from randomly distributed electrons interfere constructively and destructively in time, and the resulting radiation power scales linearly with the number of electrons. If an external laser is provided or if the synchrotron radiation becomes sufficiently strong, the transverse electric field of the radiation beam interacts with the transverse electron current created by the sinusoidal wiggling motion, causing some electrons to gain and others to lose energy to the optical field.Template:Clarify
This energy modulation evolves into electron density (current) modulations with a period of one optical wavelength. The electrons are thus bunched into little clumps, called microbunches, separated by one optical wavelength along the axis. Whereas conventional undulators would cause the electrons to radiate independently, the radiation emitted by the microbunched electrons are in phase, and the fields add together coherently.
The FEL radiation intensity grows, causing additional microbunching of the electrons, which continue to radiate in phase with each other.[7] This process continues until the electrons are completely microbunched and the radiation reaches a saturated power several orders of magnitude higher than that of the undulator radiation.
The wavelength of the radiation emitted can be readily tuned by adjusting the energy of the electron beam or the magnetic field strength of the undulators.
FELs are relativistic machines. The wavelength of the emitted radiation, , is given by [8]
or when the wiggler strength parameter K, discussed below, is small
where is the undulator wavelength (the spatial period of the magnetic field), is the relativistic Lorentz factor and the proportionality constant depends on the undulator geometry and is of the order of 1.
This formula can be understood as a combination of two relativistic effects. Imagine you are sitting on an electron passing through the undulator. Due to Lorentz contraction the undulator is shortened by a factor and the electron experiences much shorter undulator wavelength . However, the radiation emitted at this wavelength is observed in the laboratory frame of reference and the relativistic Doppler effect brings the second factor to the above formula. Rigorous derivation from Maxwell's equations gives the divisor of 2 and the proportionality constant. In an x-ray FEL the typical undulator wavelength of 1 cm is transformed to x-ray wavelengths on the order of 1 nm by ≈ 2000, i.e. the electrons have to travel with the speed of 0.9999998c.
Wiggler strength parameter K
K, a dimensionless parameter, tells the wiggler strength as the relationship between the length of a period and the radius of bend,[9]
Quantum effects
In most cases, the theory of classical electromagnetism adequately accounts for the behavior of free electron lasers.[10] For sufficiently short wavelengths, quantum effects of electron recoil and shot noise may have to be considered.[11]
Large facilities required
Today, a free-electron laser requires the use of an electron accelerator with its associated shielding, as accelerated electrons can be a radiation hazard, if not properly contained. These accelerators are typically powered by klystrons, which require a high voltage supply. The electron beam must be maintained in a vacuum which requires the use of numerous vacuum pumps along the beam path. While this equipment is bulky and expensive, free-electron lasers can achieve very high peak powers, and the tunability of FELs makes them highly desirable in many disciplines, including chemistry, structure determination of molecules in biology, medical diagnosis, and nondestructive testing.
X-ray laser without mirrors
The lack of suitable mirrors in the extreme ultraviolet and x-ray regimes prevents the operation of a FEL oscillator; consequently, there must be suitable amplification over a single pass of the electron beam through the undulator to make the FEL worthwhile. X-ray free electron lasers use long undulators. The underlying principle of the intense pulses from the X-ray laser lies in the principle of self-amplified stimulated emission (SASE), which leads to the microbunching of the electrons. Initially all electrons are distributed evenly and they emit incoherent spontaneous radiation only. Through the interaction of this radiation and the electrons' oscillations, they drift into microbunches separated by a distance equal to one radiation wavelength. Through this interaction, all electrons begin emitting coherent radiation in phase. In other words, all emitted radiation can reinforce itself perfectly whereby wave crests and wave troughs are always superimposed on one another in the best possible way. This results in an exponential increase of emitted radiation power, leading to high beam intensities and laser-like properties.[12] Examples of facilities operating on the SASE FEL principle include the Free electron LASer (FLASH) in Hamburg, the Linac Coherent Light Source (LCLS) at the SLAC National Accelerator Laboratory, the European x-ray free electron laser (XFEL) in Hamburg, the SPring-8 Compact SASE Source (SCSS), the SwissFEL at the Paul Scherrer Institute (Switzerland) and, as of 2011, the SACLA at the RIKEN Harima Institute in Japan.
Self seeding
One problem with SASE FELs is the lack of temporal coherence due to a noisy startup process. To avoid this, one can "seed" an FEL with a laser tuned to the resonance of the FEL. Such a temporally coherent seed can be produced by more conventional means, such as by high-harmonic generation (HHG) using an optical laser pulse. This results in coherent amplification of the input signal; in effect, the output laser quality is characterized by the seed. While HHG seeds are available at wavelengths down to the extreme ultraviolet, seeding is not feasible at x-ray wavelengths due to the lack of conventional x-ray lasers. In late 2010, in Italy, the seeded-FEL source FERMI@Elettra [13] has started commissioning, at the Sincrotrone Trieste Laboratory. FERMI@Elettra is a single-pass FEL user-facility covering the wavelength range from 100 nm (12 eV) to 10 nm (124 eV), located next to the third-generation synchrotron radiation facility ELETTRA in Trieste, Italy. The advent of femtosecond lasers has revolutionized many areas of science from solid state physics to biology. This new research frontier of ultra-fast VUV and X-ray science drives the development of a novel source for the generation of femtosecond pulses.
In 2012, scientists working on the LCLS overcame the seeding limitation for x-ray wavelengths by self-seeding the laser with its own beam after being filtered through a diamond monochromator. The resulting intensity and monochromaticity of the beam are unprecedented and will allow new experiments to be conducted involving manipulating atoms and imaging molecules. Other labs around the world are incorporating the technique into their equipment.[14][15]
Medical uses
Research by Dr. Glenn Edwards and colleagues at Vanderbilt University's FEL Center in 1994 found that soft tissues like skin, cornea, and brain tissue could be cut, or ablated, using infrared FEL wavelengths around 6.45 micrometres with minimal collateral damage to adjacent tissue.[16][17] This led to further research and eventually surgeries on humans, the first ever using a free-electron laser. Starting in 1999, and using the Keck foundation funded FEL operating rooms at the Vanderbilt FEL Center, Dr. Michael Copeland and Dr. Pete Konrad of Vanderbilt performed three surgeries in which they resected meningioma brain tumors.[18] Beginning in 2000, Dr. Karen Joos and Dr. Louise Mawn performed five surgeries involving the cutting of a window in the sheath of the optic nerve, to test the efficacy for optic nerve sheath fenestration.[19] These eight surgeries went as expected with results consistent with the routine standard of care and with the added benefit of laser surgery and minimal collateral damage. A review of FELs for medical uses is given in the 1st edition of Tunable Laser Applications.[20]
Since these successful results, there have been several efforts to build small, clinical lasers tunable in the 6 to 7 micrometre range with pulse structure and energy to give minimal collateral damage in soft tissue.Potter or Ceramic Artist Truman Bedell from Rexton, has interests which include ceramics, best property developers in singapore developers in singapore and scrabble. Was especially enthused after visiting Alejandro de Humboldt National Park. At Vanderbilt, there exists a Raman shifted system pumped by an Alexandrite laser.[21]
At the 2006 annual meeting of the American Society for Laser Medicine and Surgery (ASLMS), Dr. Rox Anderson of the Wellman Laboratory of Photomedicine of Harvard Medical School and Massachusetts General Hospital reported on the possible medical application of the free-electron laser in melting fats without harming the overlying skin.[22] It was reported that at infrared wavelengths, water in tissue was heated by the laser, but at wavelengths corresponding to 915, 1210 and 1720 nm, subsurface lipids were differentially heated more strongly than water. The possible applications of this selective photothermolysis (heating tissues using light) include the selective destruction of sebum lipids to treat acne, as well as targeting other lipids associated with cellulite and body fat as well as fatty plaques that form in arteries which can help treat atherosclerosis and heart disease.[23]
Military uses
FEL technology is being evaluated by the US Navy as a good candidate for an anti aircraft and missile directed-energy weapon. Significant progress is being made in raising FEL power levels (the Thomas Jefferson National Accelerator Facility's FEL has demonstrated over 14 kW)[24] and it should be possible to build compact multi-megawatt class FEL weapons.[25] On June 9, 2009 the Office of Naval Research announced it had awarded Raytheon a contract to develop a 100 kW experimental FEL.[26] On March 18, 2010 Boeing Directed Energy Systems announced the completion of an initial design for U.S. Naval use.[27] A prototype FEL system has since been demonstrated with a full-power prototype scheduled by 2018.[28]
See also
- Bremsstrahlung
- Cyclotron radiation
- Electron wake
- Gyrotron
- International Linear Collider
- Synchrotron radiation
References
43 year old Petroleum Engineer Harry from Deep River, usually spends time with hobbies and interests like renting movies, property developers in singapore new condominium and vehicle racing. Constantly enjoys going to destinations like Camino Real de Tierra Adentro.
Further reading
- Madey, John, "Stimulated emission of bremsstrahlung in a periodic magnetic field". J. Appl. Phys. 42, 1906 (1971)
- Madey, John, Stimulated emission of radiation in periodically deflected electron beam, US Patent 38 22 410,1974
- Boscolo, et al., "Free-Electron Lasers and Masers on Curved Paths". Appl. Phys., (Germany), vol. 19, No. 1, pp. 46–51, May 1979.
- Deacon et al., "First Operation of a Free-Electron Laser". Phys. Rev. Lett., vol. 38, No. 16, Apr. 1977, pp. 892–894.
- Elias, et al., "Observation of Stimulated Emission of Radiation by Relativistic Electrons in a Spatially Periodic Transverse Magnetic Field", Phys. Rev. Lett., 36 (13), 1976, p. 717.
- Gover, "Operation Regimes of Cerenkov-Smith-Purcell Free Electron Lasers and T. W. Amplifiers". Optics Communications, vol. 26, No. 3, Sep. 1978, pp. 375–379.
- Gover, "Collective and Single Electron Interactions of Electron Beams with Electromagnetic Waves and Free Electrons Lasers". App. Phys. 16 (1978), p. 121.
- "The FEL Program at Jefferson Lab" [1]
- One of the biggest reasons investing in a Singapore new launch is an effective things is as a result of it is doable to be lent massive quantities of money at very low interest rates that you should utilize to purchase it. Then, if property values continue to go up, then you'll get a really high return on funding (ROI). Simply make sure you purchase one of the higher properties, reminiscent of the ones at Fernvale the Riverbank or any Singapore landed property Get Earnings by means of Renting
In its statement, the singapore property listing - website link, government claimed that the majority citizens buying their first residence won't be hurt by the new measures. Some concessions can even be prolonged to chose teams of consumers, similar to married couples with a minimum of one Singaporean partner who are purchasing their second property so long as they intend to promote their first residential property. Lower the LTV limit on housing loans granted by monetary establishments regulated by MAS from 70% to 60% for property purchasers who are individuals with a number of outstanding housing loans on the time of the brand new housing purchase. Singapore Property Measures - 30 August 2010 The most popular seek for the number of bedrooms in Singapore is 4, followed by 2 and three. Lush Acres EC @ Sengkang
Discover out more about real estate funding in the area, together with info on international funding incentives and property possession. Many Singaporeans have been investing in property across the causeway in recent years, attracted by comparatively low prices. However, those who need to exit their investments quickly are likely to face significant challenges when trying to sell their property – and could finally be stuck with a property they can't sell. Career improvement programmes, in-house valuation, auctions and administrative help, venture advertising and marketing, skilled talks and traisning are continuously planned for the sales associates to help them obtain better outcomes for his or her shoppers while at Knight Frank Singapore. No change Present Rules
Extending the tax exemption would help. The exemption, which may be as a lot as $2 million per family, covers individuals who negotiate a principal reduction on their existing mortgage, sell their house short (i.e., for lower than the excellent loans), or take part in a foreclosure course of. An extension of theexemption would seem like a common-sense means to assist stabilize the housing market, but the political turmoil around the fiscal-cliff negotiations means widespread sense could not win out. Home Minority Chief Nancy Pelosi (D-Calif.) believes that the mortgage relief provision will be on the table during the grand-cut price talks, in response to communications director Nadeam Elshami. Buying or promoting of blue mild bulbs is unlawful.
A vendor's stamp duty has been launched on industrial property for the primary time, at rates ranging from 5 per cent to 15 per cent. The Authorities might be trying to reassure the market that they aren't in opposition to foreigners and PRs investing in Singapore's property market. They imposed these measures because of extenuating components available in the market." The sale of new dual-key EC models will even be restricted to multi-generational households only. The models have two separate entrances, permitting grandparents, for example, to dwell separately. The vendor's stamp obligation takes effect right this moment and applies to industrial property and plots which might be offered inside three years of the date of buy. JLL named Best Performing Property Brand for second year running
The data offered is for normal info purposes only and isn't supposed to be personalised investment or monetary advice. Motley Fool Singapore contributor Stanley Lim would not personal shares in any corporations talked about. Singapore private home costs increased by 1.eight% within the fourth quarter of 2012, up from 0.6% within the earlier quarter. Resale prices of government-built HDB residences which are usually bought by Singaporeans, elevated by 2.5%, quarter on quarter, the quickest acquire in five quarters. And industrial property, prices are actually double the levels of three years ago. No withholding tax in the event you sell your property. All your local information regarding vital HDB policies, condominium launches, land growth, commercial property and more
There are various methods to go about discovering the precise property. Some local newspapers (together with the Straits Instances ) have categorised property sections and many local property brokers have websites. Now there are some specifics to consider when buying a 'new launch' rental. Intended use of the unit Every sale begins with 10 p.c low cost for finish of season sale; changes to 20 % discount storewide; follows by additional reduction of fiftyand ends with last discount of 70 % or extra. Typically there is even a warehouse sale or transferring out sale with huge mark-down of costs for stock clearance. Deborah Regulation from Expat Realtor shares her property market update, plus prime rental residences and houses at the moment available to lease Esparina EC @ Sengkang - Paolo Luchini, Hans Motz, Undulators and Free-electron Lasers, Oxford University Press, 1990.
External links
- Lightsources.org
- FERMI, the new FEL at the ELETTRA synchrotron in Triest
- UCSB Free-Electron Laser
- Free-Electron Laser Open Book (National Academies Press)
- The World Wide Web Virtual Library: Free-Electron Laser research and applications
- European XFEL
- PSI SwissFEL
- SPring-8 Compact SASE Source
- Electron beam transport system and diagnostics of the Dresden FEL
- The Free Electron Laser for Infrared eXperiments FELIX
- W. M. Keck Free Electron Laser Center
- Jefferson Lab's Free-Electron Laser Program
- Free-Electron Lasers: The Next Generation by Davide Castelvecchi New Scientist, January 21, 2006
- Airborne megawatt class free-electron laser for defense and security
- FERMI@Elettra Free-Electron Laser Project
- Center for Free-Electron Laser Science (CFEL)
- ↑ Template:Cite doi
- ↑ Template:Cite web
- ↑ F. J. Duarte (Ed.), Tunable Lasers Handbook (Academic, New York, 1995) Chapter 9.
- ↑ Template:Cite web
- ↑ Template:Cite doi
- ↑ Template:Cite doi
- ↑ Feldhaus et al., J. Phys. B: At. Mol. Opt. Phys. 38 (2005) S799–S819
- ↑ Template:Cite doi
- ↑ Template:Cite web
- ↑ Template:Cite doi
- ↑ Template:Cite doi
- ↑ Template:Cite web
- ↑ http://www.elettra.trieste.it/FERMI/
- ↑ Template:Cite doi
- ↑ Template:Cite web
- ↑ Glenn Edwards et al., Nature 371 (1994) 416-419
- ↑ Template:Cite web
- ↑ Glenn S. Edwards et al., Rev. Sci. Instrum. 74 (2003) 3207
- ↑ Karen M. Joos et al., in Proc. of SPIE 4611 (2002) 81-85, eds. Manns, Soederberg, and Ho.
- ↑ F. J. Duarte (Ed.), Tunable Laser Applications, 1st Ed. (Marcel-Dekker, New York, 1995) Chapter 6.
- ↑ Template:Cite web
- ↑ Template:Cite news
- ↑ Template:Cite web
- ↑ Template:Cite web
- ↑ Template:Cite web
- ↑ Template:Cite web
- ↑ Template:Cite web
- ↑ Template:Cite news